Choosing Energy Efficient Laptops Balancing Performance with Sustainability
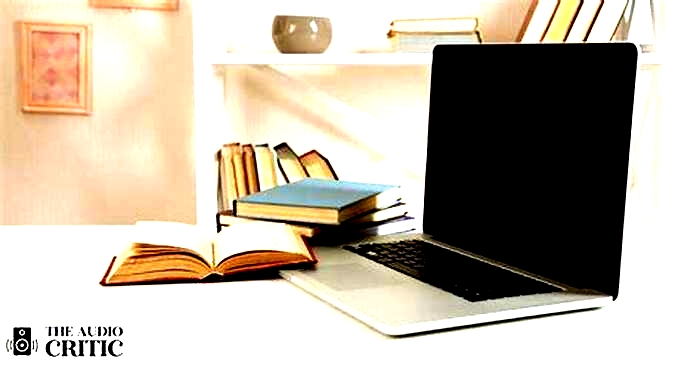
Eco Friendly Laptop
Environmental impact of a laptop
Generally, when looking at the carbon footprint of a laptop, we tend to think about their energy consumption first. A laptop that is on for around 8 hours per day emits between 44-88kg of CO2 per year, but according to Lars Meiritz, the vice-president at Gartner, only 20% of the environmental impact of a laptop arises from its use. So, what about the other 80%?
It is essential to look at the whole lifecycle of a laptop when considering its environmental impact. The resource consumption, carbon emissions, pollution, and e-waste from a laptop will be determined by factors such as:
The supply chain that brings materials to the factory
The energy and type of materials used in manufacturing
The manufacturing techniques used
Delivery to the end user
Durability and life expectancy of the laptop
End-of-life recycling potential of the laptop
MakeUseOf
With all the buzzwords being thrown around, such as "going green" and "carbon footprint," it's no wonder that sustainability has become a major global issue.
The IT world is not left out in this sustainability drive. Efforts are being put in place to make computing more environmentally friendly, hence the term "green computing."
This article will explore what green computing is, how it impacts design, and if you can balance performance with sustainability.
What Is Green Computing?
Green computing is a term that has been around for a while, but what does it mean?
In the simplest terms, green computing is a way to design, produce and use computers that minimizes the impact on our environment.
It can also be defined as any form of energy-efficient computing that includes using less power, using renewable sources for electricity, and lowering greenhouse gas emissions.
For example, green computing discourages wasteful practices such as continuously running a device when it's only being used intermittently.
What Techniques Are Involved in Green Computing?
Several techniques make for green computing. These include green manufacturing, green design, green use, and green disposal. Let's now look at these techniques in detail.
Green Manufacturing
Green manufacturing is the process of being green from the start. This involves using green materials and minimizing power consumption during production.
For a green system to be manufactured, it should have components that do not use harmful chemicals, such as halogenated flame retardants. It should also have components that meet stringent energy efficiency standards certified by an environmental organization, such as Energy Star, and green building standards, such as LEED.
Green Design
Designing green systems involves making green decisions during the design process to improve the green attributes of a system. These green decisions should consider energy efficiency, environmentally friendly materials, reduction of greenhouse gas emissions, and reduction of energy use.
Related: How Much Energy Does Your PC Use? (And Ways to Cut It Down)
Some green decisions involve assessing the life cycle of a computer during design. For example, green computing will focus on designing systems that have longer useful lives so that they aren't replaced as often. It also aims to consider how a system is used in its design.
A green computer also should be recyclable and use less energy for transportation. Creating a sustainable product cycle that starts from the point of manufacture to when its components can be recycled into a new computer.
Green Use
The "green use" technique involves using computers in the most energy-efficient way possible. For example, users should not have to deal with excessive waits when powering up a computer. The computer's speed should also fit the user's needs so that it doesn't waste battery power.
Ensuring green use also involves the proper and efficient use of green systems. For example, green computing recommends shutting down a computer when it's not in use to reduce energy waste.
Other simple practices include turning off your computer screen or even adjusting its power settings to consume less energy while still operating are also green computing techniques.
Green Disposal
Green computing disposal speaks to the recycling of computers in a responsible and environmentally friendly manner. It also means ensuring that computers can be recycled into new computers or other products.
How Do Green Computing Requirements Impact Design?
Green computing impacts design in several ways. For example, green computing requires meeting energy requirements through power management features such as sleep modes and green technology like low voltage processors, which need less electricity to operate.
Another way green computing impacts design is that green devices are recycled or reused instead of trashed, which provides green technology for other green devices.
A third way green computing impacts design is green manufacturing, which assumes efficient use of resources based on environmental impact. That means manufacturing processes should be designed to maximize the reuse and recycling of materials or should minimize any harmful effects during production or assembly.
Balancing Green Computing and Performance
Although green computing aims to reduce energy and greenhouse gas emissions, it is sometimes at odds with performance.
For example, green computing machines may implement power settings that result in slower system performance than a user expects. It also means green computers might be designed with components that are not as powerful as other consumer electronics.
However, green computing is not at odds with performance because the goal of green computing is that computers be used in the most efficient way possible, not that computers be made intentionally slow just to be sustainable.
The percentage of energy spent on actual computing compared to the energy spent on powering computers underscores this. For example, a paper published in the International Journal of Engineering Research & Technology (IJERT) revealed that out of the $250 billion spent yearly on powering computers worldwide, "only about 15% of that power is spent computingthe rest is wasted idling."
Related: Leaving Your Computer On All the Time: The Pros and Cons
The primary goal of green computing is to reduce the energy use and environmental impact of computers. Performance sometimes suffers, but green computing is not at odds with performance; green computers can still be high-performance if designed efficiently.
Green computing is more about designing high-quality computers that fit users' needs than about designing low-performance computers.
Any Trade-Offs, Even Small Ones?
Yes. There are trade-offs with green computing. The main trade-off is that green computing may require users to sacrifice some performance for green use. However, this doesn't mean a complete loss of performance or a user's ability to access high-performance features.
The difference in performance is most likely going to be negligible for most users. If you've not noticed any difference when switching from optimal mode to battery saver mode on your PC, chances are the drop in performance will be negligible for you.
Green Computing Preserves the Environment
Green computing requires green technology to reduce energy waste, green disposal practices to protect the environment, green manufacturing, making efficient use of resources, and efficient power management to save electricity. It is not at odds with performance, but it may require users to sacrifice some negligible performance for green features.
Energy Sustainability with a Focus on Environmental Perspectives
Abe JO, Popoola API, Ajenifuja E, Popoola OM (2019) Hydrogen energy, economy and storage: review and recommendation. Int J Hydrogen Energy 44(29):1507215086
Article Google Scholar
Al-Ghussain L, Samu R, Taylan O, Fahrioglu M (2020) Sizing renewable energy systems with energy storage systems in microgrids for maximum cost-efficient utilization of renewable energy resources. Sustain Urban Areas 55:102059
Google Scholar
Ali A, Mahar RB, Sheerazi STH (2019) Renewable electricity generation from food waste through anaerobic digestion in Pakistan: a mini-review. Earth Syst Environ 3:95100
Article Google Scholar
Almazroui M, en Z (2020) Trend analyses methodologies in hydro-meteorological records. Earth Syst Environ 4:713738
Article Google Scholar
Almazroui M, en Z, Mohorji AM et al (2019) Impacts of climate change on water engineering structures in arid regions: case studies in Turkey and Saudi Arabia. Earth Syst Environ 3:4357
Article Google Scholar
Al-Zareer M, Dincer I, Rosen MA (2020a) Assessment and analysis of the integrated generation IV gas-cooled fast nuclear reactor and copperchlorine cycle for hydrogen and electricity production. Energy Convers Manag 205:112387
Article Google Scholar
Al-Zareer M, Dincer I, Rosen MA (2020b) A thermal performance management system for lithium-ion battery packs. Appl Therm Eng 165:114378
Article Google Scholar
Athienitis A, OBrien W (eds) (2015) Modeling, design, and optimization of net-zero energy buildings. Ernst & Sohn, Berlin
Google Scholar
Baleta J, Mikuli H, Kleme JJ, Urbaniec K, Dui N (2019) Integration of energy, water and environmental systems for a sustainable development. J Clean Prod 215:14241436
Article Google Scholar
Ben-Alon L, Loftness V, Harries KA, DiPietro G, Hameen EC (2019) Cradle to site life cycle assessment (LCA) of natural vs conventional building materials: a case study on cob earthen material. Build Environ 160:106150
Article Google Scholar
Bielecki A, Ernst S, Skrodzka W, Wojnicki I (2020) The externalities of energy production in the context of development of clean energy generation. Environ Sci Pollut Res 27:1150611530
Article Google Scholar
BP (2016) BP statistical review of world energy 2016, 65th edn. BP, London
Google Scholar
Calise F, Dentice dAccadia M, Vicidomini M (2019) Optimization and dynamic analysis of a novel polygeneration system producing heat, cool and fresh water. Renew Energy 143:13311347
Article Google Scholar
Campana PE, Wsthage L, Nookuea W, Tan Y, Yan J (2019) Optimization and assessment of floating and floating-tracking PV systems integrated in on- and off-grid hybrid energy systems. Sol Energy 177:782795
Article Google Scholar
Chapman A, Itaoka K, Farabi-Asl H, Fujii Y, Nakahara M (2020) Societal penetration of hydrogen into the future energy system: Impacts of policy, technology and carbon targets. Int J Hydrogen Energy 45(7):38833898
Article Google Scholar
Chen S, Kharrazi A, Liang S, Fath BD, Lenzen M, Yan J (2020a) Advanced approaches and applications of energy footprints toward the promotion of global sustainability. Appl Energy 261:114415
Article Google Scholar
Chen Y, Liu A, Cheng X (2020b) Quantifying economic impacts of climate change under nine future emission scenarios within CMIP6. Sci Total Environ 703:134950
Article Google Scholar
Delavar H, Sahebi H (2020) A sustainable mathematical model for design of net zero energy buildings. Heliyon 6(1):e03190
Article Google Scholar
Dincer I, Rosen MA (2015) Exergy analysis of heating, refrigerating and air conditioning: methods and applications. Elsevier, Oxford
Google Scholar
Dincer I, Rosen MA (2021a) Thermal energy storage: systems and applications, 3rd edn. Wiley, London (in press)
Book Google Scholar
Dincer I, Rosen MA (2021b) Exergy: energy, environment and sustainable development, 3rd edn. Elsevier, Oxford
Google Scholar
Dincer I, Rosen MA, Ahmadi P (2017) Optimization of energy systems. Wiley, London
Book Google Scholar
Dragicevic AZ (2020) Concentric framework for sustainability assessment. J Clean Prod 248:119268
Article Google Scholar
Endo N, Shimoda E, Goshome K, Yamane T, Nozu T, Maeda T (2019) Construction and operation of hydrogen energy utilization system for a zero emission building. Int J Hydrogen Energy 44(29):1459614604
Article Google Scholar
Fetter S (2009) How long will the worlds uranium supplies last? Sci Am 300:84
Google Scholar
Fonseca JD, Camargo M, Commenge J-M, Falk L, Gil ID (2019) Trends in design of distributed energy systems using hydrogen as energy vector: a systematic literature review. Int J Hydrogen Energy 44(19):94869504
Article Google Scholar
Garmsiri S, Kouhi-Fayegh S, Rosen MA, Smith GR (2016) Integration of transportation energy processes with a net zero energy community using captured waste hydrogen from electrochemical plants. Int J Hydrogen Energy 41(19):83378346
Article Google Scholar
Gnanapragasam NV, Rosen MA (2017) A review of hydrogen production using coal, biomass and other solid fuels. Biofuels 8(6):725745
Article Google Scholar
Graedel TE, Allenby BR (2010) Industrial ecology and sustainable engineering. Prentice Hall, Upper Saddle River
Google Scholar
Grigoroudis E, Kouikoglou VS, Phillis YA, Kanellos FD (2019) Energy sustainability: a definition and assessment model. Oper Res Int J. https://doi.org/10.1007/s12351-019-00492-2
Article Google Scholar
Gupta A, Pradhan B, Maulud KNA (2020) Estimating the impact of daily weather on the temporal pattern of COVID-19 outbreak in India. Earth Syst Environ 4:523534
Article Google Scholar
Hachem-Vermette C, Guarino F, La Rocca V, Cellura M (2019) Towards achieving net-zero energy communities: investigation of design strategies and seasonal solar collection and storage net-zero. Sol Energy 192:169185
Article Google Scholar
Hall CAS, Lambert JG, Balogh SB (2014) EROI of different fuels and the implications for society. Energy Policy 64:141152
Article Google Scholar
Hansen K, Breyer C, Lund H (2019) Status and perspectives on 100% renewable energy systems. Energy 175:471480
Article Google Scholar
Hengst I-A, Jarzabkowski P, Hoegl M, Muethel M (2020) Toward a process theory of making sustainability strategies legitimate in action. Acad Manag J 63:246271
Article Google Scholar
IEA (2020) World Energy Statistics Database Documentation, 2020th edn. International Energy Agency, Paris
Google Scholar
IEA (2021) Explore energy data by category, indicator, country or region. International Energy Agency. https://www.iea.org/data-and-statistics/data-tables?country=WORLD&energy=Balances&year=2018. Accessed 16 Mar 2021
Jose R, Ramakrishna S (2021) Comprehensiveness in the research on sustainability. Mater Circu Econ 3:1. https://doi.org/10.1007/s42824-020-00015-x
Article Google Scholar
Karunathilake H, Hewage K, Mrida W, Sadiq R (2019) Renewable energy selection for net-zero energy communities: life cycle based decision making under uncertainty. Renew Energy 130:558573
Article Google Scholar
Kasaeian A, Bellos E, Shamaeizadeh A, Tzivanidis C (2020) Solar-driven polygeneration systems: recent progress and outlook. Appl Energy 264:114764
Article Google Scholar
Khalil A, Javed A, Bashir H (2019) Evaluation of carbon emission reduction via GCIP projects: creating a better future for Pakistan. Earth Syst Environ 3:1928
Article Google Scholar
Koohi-Fayegh S, Rosen MA (2020) A review of energy storage types, applications and recent developments. J Energy storage 27:101047
Article Google Scholar
Krishan O, Suhag S (2019) An updated review of energy storage systems: classification and applications in distributed generation power systems incorporating renewable energy resources. Int J Energy Res 43:61716210
Article Google Scholar
Kumar J, C. R., Majid, M. A. (2020) Renewable energy for sustainable development in India: current status, future prospects, challenges, employment, and investment opportunities. Energy Sustain Soc 10:2
Article Google Scholar
Kumar A, Sharma M, Thakur P, Kumar Thakur V, Rahatekar SS, Kumar R (2020) A review on exergy analysis of solar parabolic collectors. Sol Energy 197:411432
Article Google Scholar
Kutscher CF, Milford JB, Kreith F (2019) Principles of sustainable energy systems. Mechanical and aerospace engineering series, 3rd edn. CRC Press, Boca Raton (ISBN 978-0-429-93916-7)
Google Scholar
Lafta AA, Altaei SA, Al-Hashimi NH (2020) Impacts of potential sea-level rise on tidal dynamics in Khor Abdullah and Khor Al-Zubair, Northwest of Arabian Gulf. Earth Syst Environ 4:93105
Article Google Scholar
Liu Z, Yu H, Liu R, Wang M, Li C (2020) Configuration optimization model for data-center-park-integrated energy systems under economic, reliability, and environmental considerations. Energies 13:448
Article Google Scholar
Lodato C, Tonini D, Damgaard A, Astrup TF (2020) A process-oriented life-cycle assessment (LCA) model for environmental and resource-related technologies (EASETECH). Int J Life Cycle Assess 25:7388
Article Google Scholar
Lu T, Halog A (2020) Towards better life cycle assessment and circular economy: on recent studies on interrelationships among environmental sustainability, food systems and diet. Int J Sustain Dev World. https://doi.org/10.1080/13504509.2020.1734984
Article Google Scholar
Madah FA (2020) The amplitudes and phases of tidal constituents from harmonic analysis at two stations in the Gulf of Aden. Earth Syst Environ 4:321328
Article Google Scholar
Mehrjerdi H, Iqbal A, Rakhshani E, Torres JR (2019) Daily-seasonal operation in net-zero energy building powered by hybrid renewable energies and hydrogen storage systems. Energy Convers Manag 201:112156
Article Google Scholar
Mendecka B, Tribioli L, Cozzolino R (2020) Life cycle assessment of a stand-alone solar-based polygeneration power plant for a commercial building in different climate zones. Renew Energy. https://doi.org/10.1016/j.renene.2020.03.063
Article Google Scholar
Moharamian A, Soltani S, Rosen MA, Mahmoudi SMS, Bhattacharya T (2019) Modified exergy and modified exergoeconomic analyses of a solar based biomass co-fired cycle with hydrogen production. Energy 167:715729
Article Google Scholar
Morosuk T, Tsatsaronis G (2019) Advanced exergy-based methods used to understand and improve energy-conversion systems. Energy 169:238246
Article Google Scholar
Nematchoua MK, Marie-Reine Nishimwe A, Reiter S (2021) Towards nearly zero-energy residential neighbourhoods in the European Union: a case study. Renew Sustain Energy Rev 135:110198
Article Google Scholar
Park J, Kim S, Kim Y, Hong S, Suh K (2020) Evaluation of carrying capacity and sustainability of Jeju Island using Onishi Model. J Korean Soc Rural Plan 26(02):95106. https://doi.org/10.7851/ksrp.2020.26.2.095
Article Google Scholar
Pauliuk S (2020) Making sustainability science a cumulative effort. Nat Sustain 3:24
Article Google Scholar
Pillai GS, Jeevarenuka K, Hameed PS (2017) Radioactivity in building materials of Pudukkottai Geological Region, Tamil Nadu, India. Earth Syst Environ 1:4
Article Google Scholar
Qaisrani A, Umar MA, Siyal GEA et al (2018) What defines livelihood vulnerability in rural semi-arid areas? Evidence from Pakistan. Earth Syst Environ 2:455475
Article Google Scholar
Rad FM, Fung AS, Rosen MA (2017) An integrated model for designing a solar community heating system with borehole thermal storage. Energy Sustain Dev 36C:615
Article Google Scholar
Razmjoo AA, Sumper A, Davarpanah A (2020) Energy sustainability analysis based on SDGs for developing countries. Energy Sources Part A Recovery Util Environ Eff 42(9):10411056
Article Google Scholar
Rekioua D (2020) Storage in hybrid renewable energy systems. In: Hybrid renewable energy systems: optimization and power management control. Springer, Cham, pp 139172
Rezaie B, Rosen MA (2020) The energy-water-food nexus: a framework for sustainable development modeling. Energy Equip Syst 8(2):179201
Google Scholar
Rokni MM (2020) Waste to energy and polygeneration systems based on solid oxide fuel cells. ASME J Electrochem Energy Convers Storage 17(3):031011
Article Google Scholar
Rosen MA (2009) Energy sustainability: a pragmatic approach and illustrations. Sustainability 1(1):5580
Article Google Scholar
Rosen MA (2011) Economics and exergy: an enhanced approach to energy economics. Nova Science Publishers, Hauppauge
Google Scholar
Rosen MA (2012) Environment, ecology and exergy: enhanced approaches to environmental and ecological management. Nova Science Publishers, Hauppauge
Google Scholar
Rosen MA (2015) Net-zero energy buildings and communities: potential and the role of energy storage. J Power Energy Eng 3(4):470474
Article Google Scholar
Rosen MA (2017c) How can we achieve the UN sustainable development goals? Eur J Sustain Dev Res 1(2):06
Article Google Scholar
Rosen MA (2017a) Sustainable development: a vital quest. Eur J Sustain Dev Res 1(1):2
Google Scholar
Rosen MA (2017b) Enhancing renewable energy prospects via hydrogen energy systems. J Fundam Renew Energy Appl 7(5):22
Article Google Scholar
Rosen MA (2018) Issues, concepts and applications for sustainability. Glocalism J Cult Polit Innov 3:121
Google Scholar
Rosen MA, Koohi-Fayegh S (2016) Cogeneration and district energy systems: modelling, analysis and optimization. Institution of Engineering and Technology, London
Book Google Scholar
Rosen MA, Koohi-Fayegh S (2017) Geothermal energy: sustainable heating and cooling using the ground. Wiley, London
Book Google Scholar
Sadeghi S, Ghandehariun S, Rosen MA (2020) Comparative economic and life cycle assessment of solar-based hydrogen production for oil and gas industries. Energy 208:118347
Article Google Scholar
Sciubba E (2019) Exergy-based ecological indicators: from thermo-economics to cumulative exergy consumption to thermo-ecological cost and extended exergy accounting. Energy 168:462476
Article Google Scholar
Scott DS (2007) Smelling land: the hydrogen defense against climate catastrophe. Canadian Hydrogen Association, Ottawa
Google Scholar
Singh G, Das R (2020) Comparative assessment of different air-conditioning systems for nearly/net zero-energy buildings. Int J Energy Res 44:35263546
Article Google Scholar
Sliwa T, Sojczynska A, Rosen MA, Kowalski T (2019) Evaluation of temperature profiling quality in determining energy efficiencies of borehole heat exchangers. Geothermics 78:129137
Article Google Scholar
Suganthi L (2020) Sustainability indices for energy utilization using a multi-criteria decision model. Energy Sustain Soc 10:16
Article Google Scholar
Sun D, Athienitis A, DAvignon K (2019) Application of semitransparent photovoltaics in transportation infrastructure for energy savings and solar electricity production: toward novel net-zero energy tunnel design. Prog Photovolt Res Appl 27:10341044
Article Google Scholar
Tumminia G, Guarino F, Longo S, Aloisio D, Cellura S, Sergi F, Brunaccini G, Antonucci V, Ferraro M (2020) Grid interaction and environmental impact of a net zero energy building. Energy Convers Manag 203:112228
Article Google Scholar
Udayakumara EPN, Gunawardena UADP (2018) Costbenefit analysis of Samanalawewa Hydroelectric Project in Sri Lanka: an ex post analysis. Earth Syst Environ 2:401412
Article Google Scholar
United Nations (2015) Resolution adopted by the general assembly on 25 September 2015. A/RES/70/1. Seventieth session. United Nations
Veiga JPS, Romanelli TL (2020) Mitigation of greenhouse gas emissions using exergy. J Clean Prod 260:121092
Article Google Scholar
Wang C, Zhang L, Chang Y, Pang M (2021) Energy return on investment (EROI) of biomass conversion systems in China: meta-analysis focused on system boundary unification. Renew Sustain Energy Rev 137:110652
Article Google Scholar
Weisz PB (2004) Basic choices and constraints on long-term energy supplies. Phys Today 57(7):4752. https://doi.org/10.1063/1.1784302
Article Google Scholar
Were D, Kansiime F, Fetahi T et al (2019) Carbon sequestration by wetlands: a critical review of enhancement measures for climate change mitigation. Earth Syst Environ 3:327340
Article Google Scholar
World Bank Group (2021) DataBank: World Development Indicators (series GDP (Constant 2005 US$), Energy Use (kt of oil equivalent) and Population (Total). http://www.databank.worldbank.org. Accessed 19 March 2021
World Commission on Environment and Development (1987) Our common future. Oxford University Press, New York
Google Scholar
Zhang Y, Wang Y (2013) Barriers and policies analysis of Chinas building energy efficiency. Energy Policy 62:768773
Article Google Scholar
Zomer C, Custdio I, Goulart S, Mantelli S, Martins G, Campos R, Pinto G, Rther R (2020) Energy balance and performance assessment of PV systems installed at a positive-energy building (PEB) solar energy research centre. Sol Energy 212:258274
Article Google Scholar
Zvolinschi A, Kjelstrup S, Bolland O, van der Kooi HJ (2007) Exergy sustainability indicators as a tool in industrial ecology. J Ind Ecol 11:8598
Article Google Scholar